Interfacing the Brain Using Functional Near-Infrared Spectroscopy (fNIRS)
Functional Near-Infrared Spectroscopy (fNIRS) is an emerging technology to obtain information about brain activity by measuring the attenuation of light through the brain. Brain-Computer-Interfaces (BCIs) using fNIRS can be inexpensive, small and lightweight, and do not pose any risk to the user. During this project, a wearable and modular fNIRS setup is being developed for BCI applications.
Contact
Rehabilitation Engineering
Lengghalde 5
8008
Zürich
Switzerland
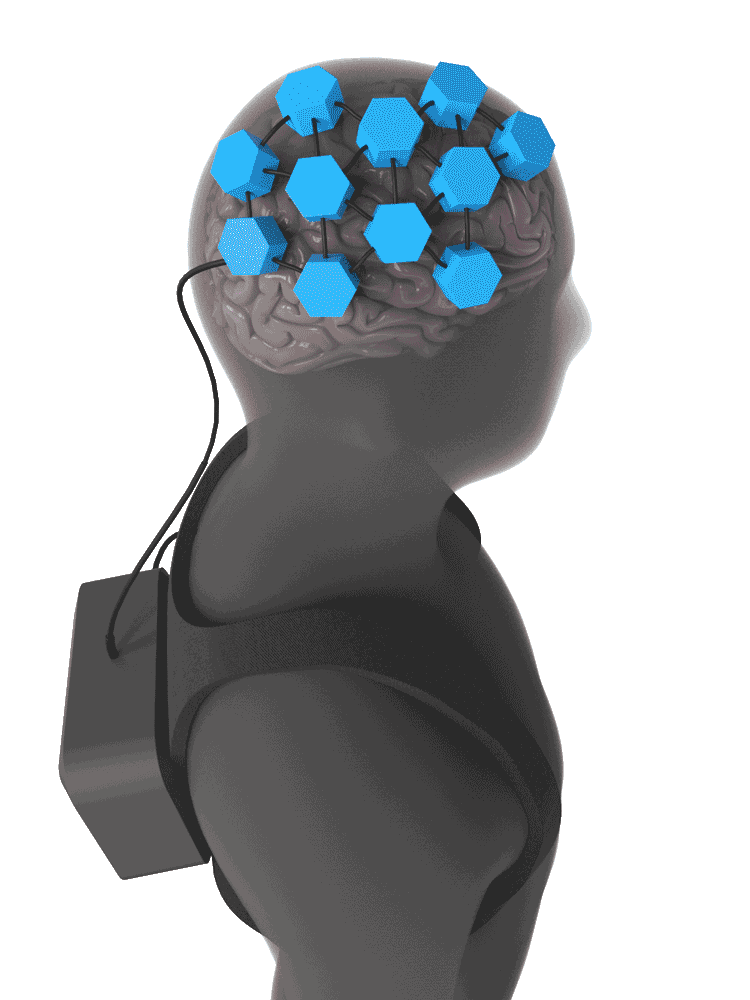
Neurological injuries such as stroke can lead to loss of limb control. Traditional therapy approaches focus on the patients’ active participation with the affected limbs. However, such active therapy concepts are inapplicable for severely impaired patients with no or very limited residual motor function. This gap could be closed by rehabilitative devices that detect the patients’ motion intention and support their movement.
The goal of this project is to develop and implement a BCI for real-time decoding of motion intention of the upper limb. The BCI is based on fNIRS, which enables safe, inexpensive and fast brain imaging. In fNIRS, brain activity is measured by monitoring changes of oxygenation in the brain (Scholkmann et al., 2014). Cerebral hemodynamics are derived by illuminating the brain with near-infrared light at multiple wavelengths and measuring its attenuation through the tissue. Changes of the hemodynamics are related to the execution and imagery of motor tasks (Zimmerman et al., 2013b). Therefore, motion intention of patients, who try to perform a motor task but are not physically able to execute it, can be detected. This information can be used to control a robotic device that can support the patients’ movement, e.g. an exoskeleton. Since near-infrared light does not pose any risk to the safety of the patients when applied correctly, we envision the application of fNIRS devices for home rehabilitation.
In a previous project, we implemented a hybrid BCI – with a commercially available fNIRS device – for the simultaneous acquisition of fNIRS signals, and biological signals from the autonomous nervous system, i.e. heart rate, breathing rate, blood pressure and skin conductance (Zimmerman et al., 2013a, 2013b; Marchal-Crespo et al., 2013). These studies motivated the construction of a new modular and wearable fNIRS system, to overcome limitations of other fNIRS devices, e.g. limited number of channels, bulkiness.
Our fNIRS setup satisfies strict requirements in terms of performance, functionality, size, modularity and safety. The setup consists of multiple small modules with a footprint of approximately 20mm x 20mm each. A module contains four LEDs with four different wavelengths and a sensor, and can operate as source or detector. A microcontroller on each module guarantees fast and modular operation of the setup. The core of the novel setup is a Silicon Photomultiplier (SiPM), which is a highly sensitive light sensor with a low-voltage operation and a relatively low price (Zimmerman et al., 2013c). The large dynamic range of SiPMs allows measurements at both very short and long source-detector-separations (corresponding to strong and weak light intensities, respectively). By including very short source-detector-separations, undesired disturbances, e.g. arterial pulse, can be better removed. The new fNIRS setup will be used to investigate algorithms for real-time decoding of brain activity. Furthermore, the combination of fNIRS with a hand exoskeleton is planned in the near future.
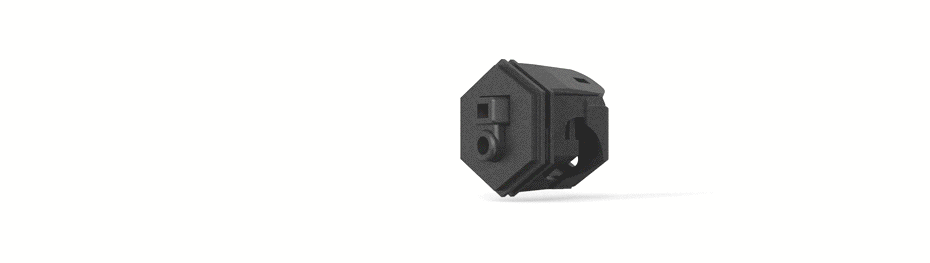
Funding
- ETH Zurich research grant CH1-02 09-3 "Cortically-Driven Assistance Adaptation during Sensorimotor Training"
- Strategic Japanese-Swiss Cooperative Research Program on "Medicine for an Aging Society"
- ETH Zurich Foundation